‘Expansion microscopy’ turns ten: how a tissue-swelling method brought super-resolution imaging to the masses

A decade in, expansion microscopy is unlocking insights across biology and medicine
In Ed Boyden’s laboratory, new students and postdocs are issued with the essential kit of their trade: micropipettes, a notebook, a computer — and a flashlight. From bicycle beacons to camping lamps, every lab member has a portable light on their bench to visualize the gossamer brain-tissue samples in their lab dishes.
These are not ordinary specimens. The tissues have been swollen 100-fold in volume — akin to inflating a hockey puck to the size of a curling stone. In the process, they become nearly translucent, like wispy jellyfish in a sea of buffer.
To the naked eye, these samples are perceptible only when lit at an oblique angle — hence the lights. Under the microscope, however, the specimens are sharply defined, revealing cellular features that can be seen only with the most sophisticated microscopes.
Boyden, a neuroscientist and biological engineer at the Massachusetts Institute of Technology (MIT) in Cambridge, first described1 the tissue-enlarging technique in January 2015 — ten years ago this week — in a paper succinctly titled ‘Expansion microscopy’.
In the decade since, at least 700 primary-research papers have used expansion microscopy or ‘ExM’, according to a running tally maintained by Boyden. Along the way, researchers around the world have refined the technique to be more versatile and fit-for-purpose than the original, or integrated it with other state-of-the-art methods to probe the function and spatial organization of proteins, nucleic acids and other biomolecules.
ExM enlarges tissues ‘isotropically’, that is, uniformly in all directions, much as an image on an inflated balloon remains in proportion and free from distortion. In so doing, the technique effectively extends the resolving power of conventional microscopes past the diffraction limit — typically around 200 nanometres, at which point fine features become indistinguishable.
Super-resolution microscopy broke this barrier long before ExM came along, but it relies on complex and costly instruments. ExM provides comparable detail using standard equipment, making nanoscale imaging available to anyone with a fluorescence microscope. “It’s the super-resolution technique for poor people,” says Anne-Sophie Hafner, a cellular neurobiologist at Radboud University in Nijmegen, the Netherlands.
The method is incompatible with live-cell imaging, and it lacks the atomic-level detail that only some of the priciest and most sophisticated technologies can offer. But ExM is steadily closing the gap. Recent innovations have allowed researchers to glimpse molecular complexes and their component parts with nearly single-nanometre precision.
“We can actually see the individual molecules,” says Silvio Rizzoli, a neuroscientist and nanoscale specialist at the University Medical Center Göttingen in Germany. “Expansion microscopy gives you opportunities that aren’t available with anything else.”
‘Kind of magical’
Boyden first started exploring the idea of expanding tissue samples for nanoscale imaging as far back as 2007, and he has the scribbled notes from those brainstorming sessions to prove it.
Brian Chow, a former postdoc in Boyden’s lab who was part of those early discussions, recalls thinking: “It’s so crazy, this just might work.” But when he tried a few materials that were lying around the lab, none fit the bill.
Chow, who is now at the health-care-investing firm Deerfield in New York City, moved on to other projects. But five years later, graduate students Fei Chen and Paul Tillberg took another stab at it. Their breakthrough came with the identification of a ‘smart’ gel, which had been described decades earlier2. Its chain-like polymers could inflate and distend to more than four times their original size in each direction when soaked in liquid.
Late one night in 2012, in the windowless basement of Boyden’s MIT lab, Chen and Tillberg immersed a brain slice in a bath of the gel’s building blocks, added chemicals to trigger gel formation and placed the sample in water. As the clock ticked past midnight, they watched and waited.
It “was kind of magical”, recalls Chen, who now runs a spatial genomics laboratory at the Broad Institute of MIT and Harvard in Cambridge. “We could literally see the brain expand before our eyes.”
As the Sun rose over the nearby Charles River, Tillberg, who continues to advance ExM at the Howard Hughes Medical Institute’s Janelia Research Campus in Ashburn, Virginia, went to bed thinking: “Okay, this is actually going to work.”
The researchers honed their technique over the next year, settling on a four-step protocol that remains the cornerstone of ExM to this day. Using it, Boyden’s team imaged synaptic connections in the mouse brain, achieving a resolution of around 70 nanometres.
Since then, the research community has incorporated changes such as different gel chemistries, reaction conditions and swelling procedures that have collectively increased sample expansion and pushed resolution below 20 nanometres. But just as the four key ingredients in beer — barley, water, hops and yeast — can produce a remarkable diversity of tipples, the core steps of ExM have remained largely constant, even as the methodology has advanced and evolved (see ‘Big brains’).
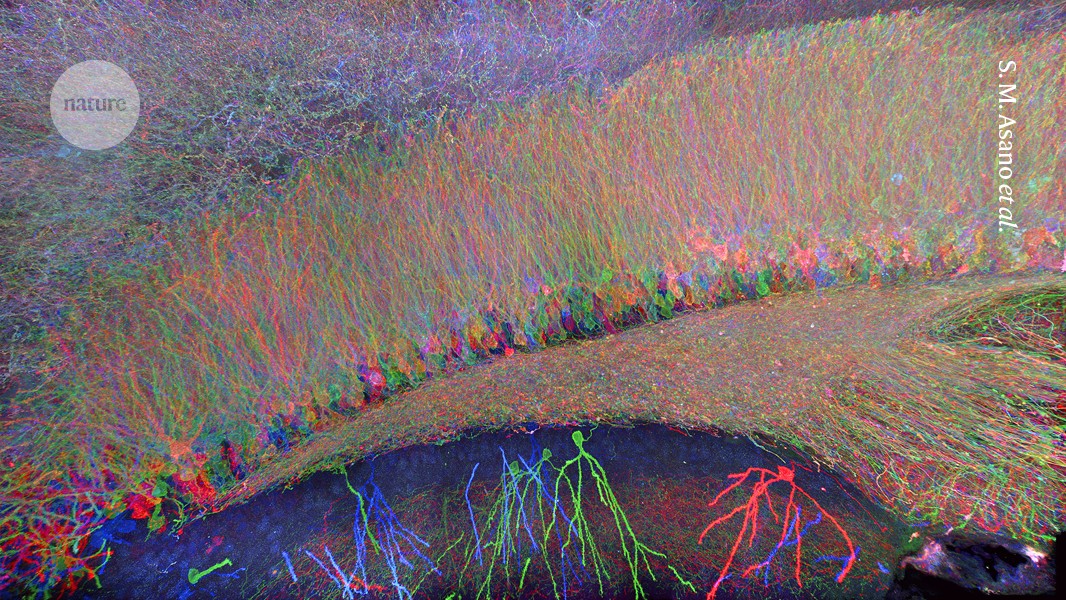
Source: A.T. Wassie et al. Nature Methods 16, 33–41 (2019)
Bigger picture biology
The first step involves fixing the sample and then applying a type of ‘anchor’ to tether molecules of interest, such as proteins, to the gel scaffold. Next, the sample is infused with the building blocks of the smart gel — Chen and Tillberg used the same absorbent material as is found in baby nappies. These precursors polymerize and link with the anchors to form a dense network that permeates each cell.
The sample is then ‘softened’ with enzymes, detergents or heat, rendering it pliable for step four: with the addition of water, the tissue gradually swells and becomes transparent. With the help of fluorescent probes, this reveals intricate molecular details when the samples are finally examined under the microscope.
The entire process generally takes a day or two, with step-by-step protocols available at websites such as expansionmicroscopy.org, which Boyden has curated for the past decade. Leaders in the field also regularly host hands-on workshops — mostly in Europe and the United States, but, over the past few years, also in parts of the world that historically have had limited access to advanced imaging equipment.
“I want to remove technical barriers,” says Xiaoyu Shi, a cell biophysicist at the University of California, Irvine, who co-directed one such workshop in Uruguay. “I want this method to reach any corner in the biology field, so people can use it and, even with little money, build up their imaging capacities.”
Another workshop took place last year in Ghana. Here, cell biologist Yaw Aniweh at the West African Centre for Cell Biology of Infectious Pathogens in Accra has been using ExM to study structural adaptations in clinical isolates of malaria parasites and other parasites endemic to the region. “In a typical resource-limited setting like ours, nobody has an electron microscope,” Aniweh says. “Expansion is the best tool we have.”
Adaptable innovation
As that tool has gained traction, researchers have embraced it with creativity, crafting adaptations to address an array of challenges. “Here’s one of the beautiful things about expansion: not only can anybody do it, you can also tweak it yourself,” says Boyden.
“The core philosophy remains the same,” he adds, “but each of those steps can be modified to your heart’s content.”
Joshua Vaughan, a bioimaging researcher at the University of Washington in Seattle, recalls reading the initial ExM report with a mix of excitement and scepticism. “Either this is a huge deal and it’s going to change the way lots of people do things,” he remembers thinking, “or there’s some kind of a trick and it’s too good to be true.”
It proved to be the former. “I couldn’t find a flaw with it,” Vaughan says.
But he was able to make it better by streamlining the procedure to use readily available fluorescent proteins and antibodies to anchor the gel matrix3 instead of the more complex, custom probes from the original report. Independently, and around the same time, Boyden’s team4 and a separate group5 reported simplified protocols of their own.
Word spread quickly, including through social media, on which it caught the attention of Paul Guichard and Virginie Hamel. The structural cell biologists have dedicated their careers to teasing apart the intricate architecture of centrioles, barrel-shaped organelles involved in cell division and organization. But when they finished their postdocs and started a joint lab group at the University of Geneva in Switzerland in 2015, they no longer had access to the multimillion-dollar equipment they needed to visualize nanoscale cellular structures.

Nuclear pores seen using a form of ultrastructure expansion microscopy (right) compared with no expansion (left) and a type of super-resolution microscopy (middle).Credit: V. Louvel et al./Nature Commun.
Eager to find a suitable workaround, they tested the existing ExM protocols but found that the centrioles expanded unevenly, compromising structural accuracy. Undeterred, Guichard and Hamel refined the gel chemistry and optimized the fixation and labelling processes to preserve the organelle’s intricate form6. This optimized ‘ultrastructure’ variant, termed U-ExM, enabled the researchers to construct a mechanistic model of human centriole assembly7. And it proved equally adept at mapping the molecular architecture of mitochondria, microtubules and single-celled microorganisms.
In the case of the latter, U-ExM uncovered a treasure trove of strange and unexpected cytoskeletal shapes in lab-grown microalgae: twisting spirals, branching filigrees and more. Hamel, Guichard and their colleagues are now extending these studies to water samples collected along coastlines from the Baltic to the Mediterranean Sea, aiming to compile an atlas of cytoskeletal diversity with which to track the impact of climate change on planktonic communities.
“It’s a new world,” Guichard says. “There are so many structural organizations we have never seen before.”
Singular focus
Also never seen before — at least, not with such straightforward tools — are the nanoscale details that are being revealed thanks to an advance8 reported in October by Rizzoli, Ali Shaib, who is also at Göttingen, and their colleagues.
At the heart of the one-step nanoscale expansion (ONE) approach is an analytical technique that captures thousands of images of the sample as fluorescent signals from tagged protein fragments flicker on and off. These images are then processed by an artificial-intelligence algorithm, which reconstructs the sample’s molecular architecture with near-atomic resolution.
When applied to a neurotransmitter receptor in the brain, ONE microscopy unveiled features that had eluded even the most advanced alternative experimental techniques. “It is now well beyond the capacity of conventional super-resolution,” Shaib says.
Enjoying our latest content?
Login or create an account to continue
- Access the most recent journalism from Nature's award-winning team
- Explore the latest features & opinion covering groundbreaking research
or
Sign in or create an accountNature 637, 752-754 (2025)
doi: https://doi.org/10.1038/d41586-025-00059-6
This story originally appeared on: Nature - Author:Elie Dolgin