Brain drugs can now cross the once impenetrable blood–brain barrier

New technologies to shuttle drugs into the brain are showing promise in Alzheimer’s disease, cancer and more
Daiza Gordon watched her two younger brothers die when they were adolescents. They had Hunter syndrome, a rare, incurable disease — predominantly affecting boys — in which a gene for an important enzyme is missing. Guilt compounded her grief when her attempts to resuscitate her youngest brother failed. She was just 19 years old.
Gordon went on to discover how merciless genetics can be. Her own three sons were all born with the condition. When her two eldest hit their second birthdays, the symptoms started to emerge: a thickening of facial features, loss of language, hearing and movement and other impacts to mental and physical development.
But she sees hope for her sons that was denied to her brothers. Her children are enrolled in a clinical trial testing a technology to carry a replacement for the missing enzyme, called iduronate-2-sulfatase (IDS), into the brain. Early results indicate improvement in some of the condition’s cognitive and physical symptoms. Gordon’s eldest sons are no longer deaf and they have started to run around. They are meeting developmental milestones she’d never dared to hope for. Her two-year-old, who started the therapy when he was just three months old, is showing none of the early symptoms. “When I look at them, I realize they have a chance of an actual future,” says Gordon.
Regular infusions of replacement IDS has been the standard of care for the past two decades, and it protects important organs such as the liver and kidneys from damage. But without help, the large enzyme can’t make it through the protective barrier that separates the blood from one of the most important organs — the brain.
For Gordon’s children, that help comes from an innovative molecular transport system, a chemical tag attached to IDS that shuttles it through the tightly joined cells that make up the blood–brain barrier. Several such shuttles, which take advantage of natural transport systems in the brain, are now being developed. With the ability to move large biological drugs — including antibodies, proteins and the viruses used in gene therapy — these shuttles promise to revolutionize neuropharmacology. And that’s not just for rare diseases such as Hunter syndrome, but also for cancer, Alzheimer’s disease and other common brain disorders.
The field is in its infancy, and much is left to discover about how to target large therapeutic molecules to the exact spots in the brain where they’re needed, says James Gorman, principal investigator of the Wyss Institute Brain Targeting Program at Harvard University in Boston, Massachusetts. Nevertheless, the excitement is palpable. “It seems like every major company in this space has a programme to develop brain shuttles,” he says.
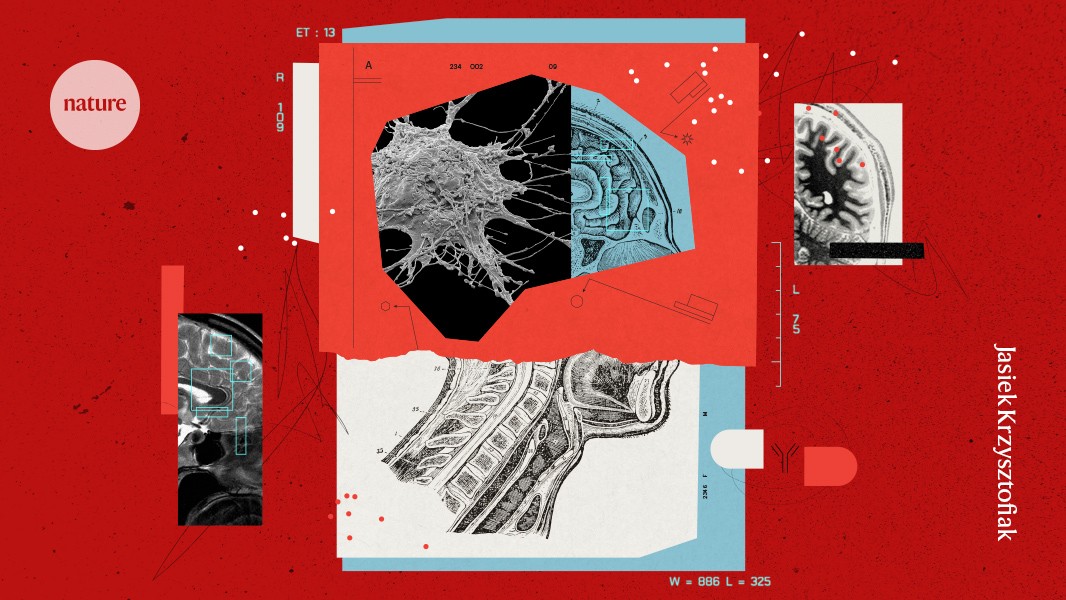
Dazia Gordon’s three sons receive regular injections of an enzyme that has been designed to cross the blood–brain barrier.Credit: Daiza Gordon
Hitting the wall
Floating in its protective sac in a sea of cerebrospinal fluid, the human brain is a highly demanding organ. Its hefty metabolic and other needs are supplied by around 650 kilometres of large and small blood vessels that are lined with a layer of tightly packed endothelial cells. This lining forms the blood–brain barrier that keeps out toxic molecules while allowing passage to those that the brain requires. Oxygen and other small, fat-soluble molecules simply diffuse through. But some molecules such as iron and glucose require specialized transporters that are embedded in the endothelial cells.
Pharmaceutical developers have generally tried to keep drugs for the brain small and fat-soluble enough to pass through the blood–brain barrier unhindered. Some small synthetic drugs take advantage of transporters — for example, the Parkinson’s disease drug levodopa hitches a ride on a transporter that normally grants access to an amino acid.
But a different approach is required for large biological drugs, which offer fresh ways of interrupting disease processes — such as breaking up clumps of proteins, replacing missing enzymes and fixing or replacing malfunctioning genes. These medicines don’t cross easily.
In the past four years, the US Food and Drug Administration (FDA) has approved several antibodies that target the amyloid proteins that form brain plaques in Alzheimer’s disease. They are the first therapies to tackle the disease’s underlying pathology, but less than 0.1% of an intravenous dose gets through the blood–brain barrier.
“With so little antibody getting into the brain, high doses have to be given — a waste of material, and a likely source of side effects that are occasionally serious,” says neurobiologist Dag Sehlin at Uppsala University in Sweden.
How this small amount of antibody penetrates the brain’s fortress had been a mystery until the past year or so. Research now indicates that its route is indirect, seeping into the cerebrospinal fluid from blood vessels in the brain sac where the barrier is somewhat less tight1.
This route is far from optimal. When small molecules cross the blood–brain barrier directly, they flow into the tiniest of capillaries that serve brain cells. When large molecules enter the cerebrospinal fluid, they tend to hang around the outside of the arterial blood vessels that carried them there, where a lot of the brain’s amyloid also settles. That presents two problems. The antibodies don’t distribute to deeper parts of the brain and they can attack the amyloid alongside the blood vessels, causing inflammation and small bleeds that can be life-threatening.
Scientists have been searching for better, direct routes into the brain for more than 25 years, says Azad Bonni, who heads neuroscience research and development at pharmaceutical company Roche in Basel, Switzerland, “but things are only now really taking off”.
The most advanced approach attempts to exploit the system involved in ensuring the brain’s supply of iron, which is required for many important enzymes and is carried through the blood by the protein transferrin (see ‘Crossing the divide’). This large iron-bearing molecule is shuttled across the blood–brain barrier by transferrin receptors on endothelial cells.

Source: S. Edavettal et al. Med 3, 860–882 (2022)
With enough protein-engineering skills, almost any therapeutic biological agent could be helped through the barrier by attaching it to a molecular construct that targets the receptor in the same way that transferrin does, says Bonni. The construct usually includes a small part of an antibody made to bind to the receptor.
“It took many years of research work to figure out how to make a safe brain shuttle,” says Bonni. Researchers had to ensure that it wouldn’t interfere with the normal function of the transferrin receptor; that it wouldn’t get stuck inside the endothelial cells; and that the therapeutic agent it’s shuttling still works when it reaches its target.
The brain’s hall pass
Shuttle-based therapies are under active development for an array of brain conditions. “It’s now abundantly clear that we can get these drugs across the blood–brain barrier,” says Gorman. “The big frontier facing us is how to efficiently get them where they need to go within the brain for different diseases and with different cargoes.”
Some diseases lend themselves particularly well to brain shuttles. Hunter syndrome has been one of the first to benefit. It is part of a family of rare genetic conditions known as lysosomal storage diseases. Lysosomes are subcellular waste-processing centres that contain enzymes (including IDS), which break down a cell’s waste products.
Cells consider foreign proteins, such as therapeutic IDS, as waste, and direct them straight to the lysosome, says Gorman, “which is perfect — because that is where the replacement enzyme needs to be”.
Enjoying our latest content?
Login or create an account to continue
- Access the most recent journalism from Nature's award-winning team
- Explore the latest features & opinion covering groundbreaking research
or
Sign in or create an accountNature 641, 1086-1088 (2025)
doi: https://doi.org/10.1038/d41586-025-01569-z
This story originally appeared on: Nature - Author:Alison Abbott