Why rings of RNA could be the next blockbuster drug
The commercial success of RNA vaccines for COVID-19 has revved up interest in circular RNAs as the next generation of therapies
RNA-based vaccines were the heroes of the COVID-19 pandemic. They set records for the highest-grossing drug launches in history, and their development was recognized in this year’s Nobel Prize in Physiology or Medicine. But it was long known that this technology had a key shortcoming: RNA, in its usual linear form, is short-lived. Within hours, enzymes in cells descend on the molecule, chewing it to pieces.
RNA’s fleeting nature isn’t a big problem for a vaccine: it needs to encode proteins only for a short time to trigger an immune response. But for most therapeutic applications, it would be much better to have RNA that could stick around for longer.
That’s where circular RNAs, or circRNAs, come in. Tie the ends of an RNA transcript together, and many RNA-munching enzymes have nothing to sink their teeth into. As a ring, RNA gains stability and longevity that, in theory, could increase its therapeutic potential, even at low dose levels.
“With a single delivery, you can get quite durable protein production,” says Howard Chang, a molecular geneticist at Stanford University School of Medicine in California and a scientific co-founder of Orbital Therapeutics in Cambridge, Massachusetts — one of the dozen or more biotechnology firms that are now pursuing therapeutics based on engineered circular RNA.
These biotech firms have collectively raised well in excess of US$1 billion in venture-capital funding over the past three years, and many big pharmaceutical companies are now dabbling in the technology as well. They are driven by the belief that whatever linear RNA can do, its more resilient circular counterpart can do better.
Proponents anticipate that circRNA will emerge as the preferred RNA platform for the drug industry, and could lead to products from next-generation vaccines and rare-disease treatments to anti-cancer agents and beyond. The first human trials of such medicines kicked off in August.
But circular RNAs are still a long way from unlocking a revolution, or delivering on the promise of 100 new drug programmes by the decade’s end, as one start-up firm has predicted (go.nature.com/3pwbkjx). And whether the added resilience of circRNA will enable it to outcompete other long-lasting therapeutic approaches — such as conventional gene therapies or emerging gene-editing techniques — remains an ongoing area of investigation and scientific enquiry.
“Don’t get me wrong, I think circular RNA is the shit,” says Jake Becraft, co-founder and chief executive of Strand Therapeutics, a synthetic-biology company in Boston, Massachusetts, that is using circRNA in some of its drug programmes. “But there are an incredible number of challenges that people have completely glossed over.”
A loopy idea
Researchers found the first circular RNAs in nature in 1976, when a team in Germany described in plants a series of small, virus-like RNA pathogens that take a closed, loop-shaped form1. Fifteen years later, investigators identified the molecules in human and other mammalian cells2.
Yet it would take until the 2010s for researchers to truly appreciate the extent of ring-shaped RNAs in various cell types — and to discover their multifaceted roles in directing biological activity.
For the most part, their role is to bind to regulatory molecules to mediate gene expression. But some circRNAs can also encode proteins — a function that scientists soon realized could have therapeutic potential, if only they had a way to make RNA circles from scratch.
In cells, the circles arise through an unconventional mode of messenger RNA processing known as back-splicing. Typically, RNA splicing operates much like film editing, with non-coding segments excised and the remaining coding portions joined together. But in some instances, the RNA takes an unexpected turn, folding back on itself, pinching off and forming a standalone loop.
Back-splicing requires an intricate dance between various proteins, all of which are naturally present inside cells but not readily available at the laboratory bench. So, in the early 1990s, researchers came up with two possible workarounds for creating synthetic circular RNAs.
One uses DNA bridges to hold the ends of an RNA strand together while another enzyme seals it, a process known as ligation3. The other harnesses the enzymatic properties of specialized RNA sequences themselves4,5. When two such sequences pair in a hairpin formation, they can initiate cross-joining reactions, forming a ring (see ‘RNA round-up’).
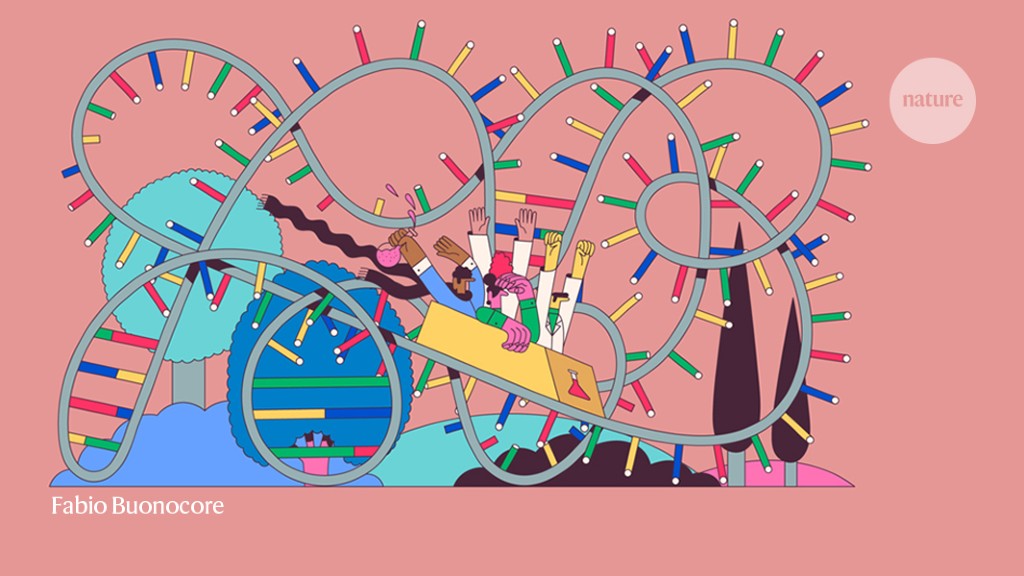
Source: Adapted from L. Santer et al. Mol. Ther. 27, 1350–1363 (2019)
In 1995, a group at the University of Colorado in Denver worked out how to synthesize proteins from such lab-made circles, using a specialized sequence called an IRES6. Short for internal ribosome entry site, the IRES allows the ribosome, the cell’s protein-making machine, to bind to a circular RNA transcript and start continuously churning out proteins. “The ribosome never needs to pop off,” says Grace Chen, an RNA biologist at Yale University School of Medicine in New Haven, Connecticut.
The researchers could create only short circular sequences, however — generally, only a few hundred nucleotides at most. And for the next 20 years, the field was stuck with this size limitation, making it impossible to generate the long protein-coding transcripts needed to address diseases such as cystic fibrosis or haemophilia.
Alex Wesselhoeft set out to change that.
Doing the rounds
Working as a graduate student at the Massachusetts Institute of Technology (MIT) in Cambridge, Wesselhoeft started with the ligation method, but it proved to be inefficient for larger circles. As with tying a long ribbon into a bow, the challenges of handling the ends become more pronounced as the RNA increases in size, making it harder to achieve a well-formed loop structure.
Switching to the self-splicing approach, Wesselhoeft designed RNA strands that had protein-coding areas and IRES elements flanked by self-splicing sequences, as others had done before. But in collaboration with bioengineers Piotr Kowalski, now at University College Cork in Ireland, and Dan Anderson at MIT, he also added complementary bits of RNA and spacer sequences in various spots along the molecule, which helped to stabilize the hairpin structure that enables circularization.
This made all the difference. The synthetic RNA could now circularize effectively, even with longer sequences7. Experiments in mice showed that these circRNAs could elicit protein production for days, whereas linear mRNA yielded protein for only about 24 hours8. The circularization technique, first reported in 2018, quickly established itself as the field’s go-to method for in vitro circRNA synthesis.
“It really made things more efficient,” says Jason Rausch, an RNA biochemist at the US National Cancer Institute in Frederick, Maryland, who adopted the technique for his own circRNA project.
In 2019, Wesselhoeft and Anderson, together with Raffaella Squilloni, a biotech entrepreneur, founded a company to commercialize the platform. Originally called Oroboros Bio after the mythical serpent that forms a ring to devour its own tail, the start-up later changed its name to Orna Therapeutics.
As head of molecular biology at Orna, Wesselhoeft continued to refine and optimize the process. Eventually, he crafted a super-long circRNA encoding dystrophin, the massive protein that is deficient in Duchenne muscular dystrophy. The transcript contained nearly 12,000 nucleotides. Dystrophin, Wesselhoeft says, “is about the largest thing in the human genome that you’d ever want to express”.
Orna was not the only start-up honing its circularization craft, however, and others have taken different approaches to building circRNAs.
Some companies pack the instructions for generating circRNA into viral vectors or DNA cassettes, and then let the splicing happen in the cell. “Our virus does the dirty work for us, here,” says Aravind Asokan, a synthetic virologist at Duke University in Durham, North Carolina, and co-founder of Torque Bio in Research Triangle Park, North Carolina. “It’s actually making the circle from within the nucleus.”
Another company, Chimerna Therapeutics in New York City, is using genetically engineered bacteria to make its circRNA in a way that chief executive Brian Pickering says is “a huge cost-saver and time-saver” compared with fully lab-generated molecules.
But most are building on Wesselhoeft’s protocol. In August, a team at biotech firm Rznomics in Seongnam, South Korea, described a system for circularizing RNA that avoids leaving any unwanted self-splicing sequences in the circRNA9. Researchers at two companies in China — CirCode Biomedicine in Shanghai and Suzhou CureMed Biopharma Technology — separately posted preprints last year that outline similar approaches10,11.
“The final circRNA contains only the coding region and the IRES”, and doesn’t have any unwanted sequences or artefacts, says Chijian Zuo, head of research and development at Suzhou CureMed.
Circular argument
Removing these extra bits of sequence might ensure that circRNAs do not provoke undesired immune responses, which could undermine their therapeutic efficacy. That’s important if you want a therapy that could be given again and again over a lifetime, says Thomas Kirkegaard Jensen, co-chief executive of Aloop Therapeutics in Copenhagen, a company aiming to treat rare genetic disorders with circRNA.
“We really need to think about every single element that contributes to immunogenicity and try to mitigate against it as much as we can,” he says.
But reports vary on whether circRNAs trigger immunity. Ling-Ling Chen, an RNA biologist at the Shanghai Institute of Biochemistry and Cell Biology, says, “It really depends how you make the circles.” In a paper first published online in 2021, she and her colleagues detailed how the sequences left behind by the self-splicing motifs tend to distort RNA folding, resulting in circles with irregular structures that prompt an immune reaction12.
Around the same time, however, RNA biochemist Oliver Rossbach and his colleagues at the Justus Liebig University of Giessen in Germany reported that contaminants were likely to be responsible, and that reactions could be minimized or eliminated with the right purification steps13. “It’s got to be super clean,” Rossbach says.
In some contexts, however, immune reactivity can be desirable. For vaccines — both for cancer and infectious diseases — provoking the immune system can spur production of antibodies and T cells. That’s what Wensheng Wei, a genome-editing scientist at Peking University in Beijing, and his colleagues discovered when they developed a vaccine for the coronavirus SARS-CoV-2 using circRNA.
In mouse and monkey experiments, the circRNA vaccine prompted production of more virus-destroying antibodies than did a linear vaccine made of the same type of modified mRNA in approved COVID-19 vaccines, and led to more potent T-cell responses14. As an added bonus, the circRNA jab was more stable than mRNA at ambient temperatures, potentially allowing the vaccine to be stored and transported without cold-chain requirements.
A company that Wei founded, Beijing-based start-up Therorna, is now testing this vaccine in people. The trial is thought to be the first to test a synthetic circRNA medicine in humans. Next year could see a handful of others enter the clinic, including a cancer therapeutic from Suzhou CureMed that encodes an immune-stimulating molecule called interleukin-12.
Orna is also gearing up to begin trials in 2024, of a circRNA that reprograms immune cells to attack blood cancer. At a conference in May, Orna scientists showed that this circRNA candidate, even when administered at low doses, could eradicate tumours in a mouse model of leukaemia, without any of the complex cell engineering or intense preparatory drug regimens required for most comparable immune therapies available today.
Revolving priorities
Synthetic circRNA can do more than encode therapeutic proteins. When folded into certain shapes, the molecules can act like antibodies and bind directly to targets, creating a type of drug known as an aptamer. They can capture and sequester different kinds of regulatory molecule, effectively purging them from the cellular environment.
They can also function as ‘antisense agents’ that bind to gene transcripts and block or alter their expression. Furthermore, they can serve as guide molecules for RNA-editing applications, directing specialized enzymes towards mutated disease-gene transcripts that are in need of correction. All of these applications are actively being explored by various start-up companies.
But the largest investments are in protein expression, and much early research and development has focused on finding ways to improve the production efficiency of the circRNA platform. “If you optimize enough, it can be more robust,” says CirCode co-founder Zefeng Wang, an RNA biologist at the Partner Institute for Computational Biology in Shanghai.
For most research teams, this process starts with the IRES. In results first published last year, for example, Chang and his colleagues systematically characterized dozens of IRES elements from various viruses, discovering many that enabled more-robust protein expression than the ones commonly used in the scientific community. By fine-tuning a few other design elements, they also successfully amplified the circRNA’s productivity by several hundred-fold, resulting in sustained protein levels for days15.
“The improvements are modular,” Chang says. “They kind of stack on top of each other.”
But progress has come in fits and starts — and, as the field has matured, it has faced some growing pains.
In June, news surfaced about data-integrity issues at Laronde, one of the most lavishly funded start-ups in the field. The company, based in Somerville, Massachusetts, was compelled to abandon one its most advanced drug programmes as a result.
According to Laronde’s chief executive, John Mendlein, those setbacks are “not indicative of the company today, nor of the science or the people”. Nevertheless, the incident has cast doubt among certain observers about the potential of circRNA.
And circles and lines are not the only ways of encoding RNA-based therapeutics. Many researchers argue that even longer-lasting technologies, such as self-amplifying RNAs that can copy themselves in the cell, will be necessary to transform synthetic RNA into a viable treatment for many chronic conditions.
But Wesselhoeft, who is now director of RNA therapeutics at the Mass General Brigham Gene and Cell Therapy Institute in Cambridge, remains bullish on circular molecules. Even with all of the success of linear mRNA vaccines, he sees circRNA as the future. “It’s going to be the RNA therapeutics technology of choice,” he says.
Nature 622, 22-24 (2023)
doi: https://doi.org/10.1038/d41586-023-03058-7
This story originally appeared on: Nature - Author:Elie Dolgin