How do you make salty water drinkable? The hunt for fresh solutions to a briny problem

Unconventional methods for desalination could create more drinking water, help many industries to deal with problematic brines and increase lithium supplies for batteries
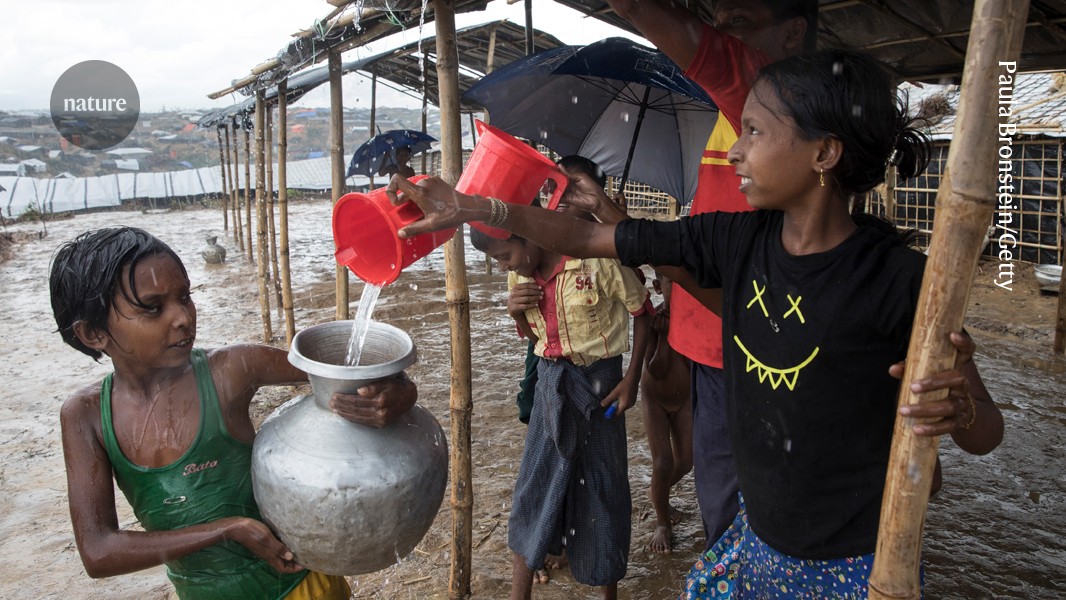
High levels of sodium in drinking water have been linked to increased rates of pre-eclampsia in Bangladesh.Credit: Paula Bronstein/Getty
People have been separating salt from water for millennia, harvesting both salt and fresh drinking water from salty seawater. But there are limits to what can be done — sometimes with drastic consequences. When people in ancient Mesopotamia couldn’t work out how to desalinate their irrigation water and prevent salts from accumulating in their soils, their society collapsed. “It’s kind of the world’s oldest, most boring, but serious problem,” says Sujay Kaushal, a hydrologist at the University of Maryland in College Park.
This problem is now growing more pressing, as salinity levels creep up in fresh waters for a slew of reasons. Rising sea levels are pushing salt into coastal groundwaters, while excessive groundwater extraction in other places is drawing deeper, saltier waters up into aquifers. And human activities — from deicing roads to washing clothes and fertilizing fields — are polluting surface waters with many kinds of salt. Last October, Kaushal and his colleagues reported that salt levels in major streams and rivers around the world are booming; some bodies of water are now several times saltier than they were a few decades ago1. Freshwater salinization is a massive global problem, not just a regional one, he says.
A second, related issue is the growing burden of problematic waste brines. A variety of industries — from oil and gas extraction to the desalination plants that produce drinking water — create salty waste waters that are costly to dispose of. “We need to do something with the brine,” says Menachem Elimelech, an environmental engineer at Yale University in New Haven, Connecticut.
On the flip side of these problems is the salt-mining industry. Hundreds of millions of tonnes of mineral salts are extracted every year from rock or briny waters. The Dead Sea is a major source of potassium; the Great Salt Lake in Utah, magnesium. Mining companies seeking supplies of lithium, a metal crucial for batteries and green technologies, are turning to brines around the world.
Researchers who sense opportunities in this field are hoping that they can extract salts from waste brines, turning a problem into a profit while squeezing out more fresh water.
To do all that, scientists are now exploring techniques to separate salt from water more efficiently, using electricity, new materials and solvents. With a wide range of brine chemistries to tackle and a host of different goals, there isn’t one “killer” technology, says Shihong Lin, an environmental engineer at Vanderbilt University in Nashville, Tennessee. “This is like a thousand different problems,” says Lin.
Super salty
It’s clear that extremely salty water isn’t healthy for humans, animals or plants. Drinking seawater, which contains about 3.5% salt, most of it sodium chloride, can shut down our kidneys and be fatal. Brackish groundwater, which contains around 0.1–1% salt, is typically desalinated before drinking. But the health impacts from less-salty water are murky. “Whether you can drink it for 30 years without any problem, not increasing any risk of disease, nobody knows,” says Lin.
There’s no agreed limit for a safe level of salt in drinking water. The World Health Organization suggests that drinking water should have sodium levels below 200 milligrams per litre (0.02%) and chloride levels below 250 mg/L (0.025%), but these guidelines are based mainly on taste. Kaushal points to a study that has linked drinking water with sodium levels of more than 0.03% to increased rates of pre-eclampsia and gestational hypertension among pregnant people in Bangladesh2. Salty water could also pose indirect harms, for instance by helping heavy metals to leach out of soils or plumbing as these metals swap places with salt in the water, says Allison Lassiter, a social scientist at the University of Pennsylvania in Philadelphia.
With salinity on the rise, specialists agree that the top priorities should be to stem salt-polluting human activities, conserve fresh water and reuse waste waters. But many researchers, including Lassiter and Kaushal, expect desalination to be increasingly needed as a buffer against freshwater scarcity.

Lithium mines in Chile spread brines over vast areas to evaporate the water. Researchers are seeking alternative, more compact methods of extracting lithium salts.Credit: Cristobal Olivares/Bloomberg/Getty
A standard method for desalination involves heating seawater to evaporate the water, then condensing the vapour; this basic principle is used today in a large number of the world’s desalination plants, especially those that dot the coasts of the Gulf in the Middle East. But this method consumes a lot of energy.
A more energy-efficient technique emerged in the 1960s, using physical pressure to force water molecules through tiny pores of a thin membrane while leaving dissolved salt ions behind. This process, called reverse osmosis, is the gold standard for desalination plants today.
The trouble is that reverse osmosis has a limit. As fresh water is extracted, the source waters get ever-saltier, making it harder and harder to continue the separation process. This is an “inescapable problem”, says Christopher Fellows, a chemist at the Saline Water Conversion Corporation (SWCC) in Jubail, Saudi Arabia. All forms of desalination leave a waste brine that needs to be managed.
Mine the brine
Some waste brines are simply put into the ocean; in California, a pipe system called the Brine Line carries waste brines that are produced more than 100 kilometres inland out to sea. In other places, the most economical solution is to inject waste brines underground, in a spot far enough away from utilized groundwater. This technique has been criticized for its potential to cause micro-earthquakes.
Alternatively, brine can be spread out in ponds to evaporate under the Sun, and the leftover salts collected — a land- and time-consuming strategy that also demands an amenable climate. A faster, more compact way to concentrate brine entails heating it and compressing the water vapour to accelerate evaporation. But this requires a staggering amount of energy, says environmental engineer Ngai Yin Yip at Columbia University in New York City, as well as expensive alloys that can withstand corrosive hot brine.
Paying for the safe disposal of brines can be exorbitant. Communities that have brackish groundwater, for example, sometimes can’t afford desalination because of the costs of brine disposal, and so must find fresh water elsewhere. Researchers who have suggested desalinating California’s Salton Sea — which is growing so salty that it threatens the wildlife living in it — are also contending with high brine-management costs.
Rather than throwing it away, some researchers are thinking about mining waste brines for minerals. For environmental engineer Jason Ren at Princeton University in New Jersey, this idea aligns better with his opinion that clean drinking water should be a human right: desalination companies, he says, should profit from selling salts, not clean water. “For many years, we’ve missed the point,” says Ren. “We focused on the water as the product; in my view, water should be a by-product of the other resources.”
Ren and others have their sights set on one particularly valuable mineral: lithium. Today, a large chunk of the world’s lithium supplies comes from natural brines in arid South America. The brine is spread out in sprawling ponds, evaporating under the Sun for many months. As researchers identify other lithium-rich brines — including waste waters from the oil and gas industry — they are realizing they need new techniques to suit places where there isn’t enough land or the right climate for evaporation ponds.

Researchers at Princeton University in New Jersey use the principle of chromatography to separate lithium from water and other salts along vertical strings.Credit: Bumper DeJesus/Princeton University
Other brines could provide an unconventional source of sodium chloride. Today, ironically, some companies use fresh water to mine salt: they puncture underground salt deposits with pipes carrying fresh water to dissolve sodium chloride. This highly pure brine is then pumped up and piped or transported to chlor-alkali plants — more than half of all chemicals produced rely on these chemical refineries.
Even if waste brines don’t contain any particularly valuable salts, water researchers have other reasons to champion the idea of mining waste brines: removing salt from brine liberates more fresh water, and the cost of disposal drops when volumes of waste brine are smaller.
Fresh solutions
In Saudi Arabia, recognizing the opportunity to bring in extra revenue while producing more fresh water, the government-owned SWCC is now building a demonstration plant to harvest sodium chloride, among other salts, from seawater desalination wastes.
The plant, scheduled to break ground at the end of this year in Haql, Saudi Arabia, uses an emerging salt-sorting technique called nanofiltration as part of a long string of processes, says Fellows. Like reverse osmosis, nanofiltration works by pushing water molecules across a membrane. But the membrane has larger pores that also allow some salt ions through: dissolved salt ions carrying only one electrical charge, such as sodium, potassium and chloride, can cross the barrier, whereas those with two or more charges, such as magnesium and calcium, stay behind. The SWCC’s key challenge is to produce sodium chloride that is pure enough for the chlor-alkali market.
The final stage at the SWCC plant entails boiling the hot brine until pure sodium chloride crystallizes. This energy-intensive step is far from ideal, says Fellows. His team has begun exploring other strategies for this stage, including freezing desalination. This approach is inspired by the fact that sea ice is composed of fresh water, even though seawater is salty. It is alluring, Fellows says, because it takes one-seventh of the energy to freeze ice-cold water as it does to evaporate boiling water. “I don’t know what’s the winning [strategy] at the moment, but it will be different for the different separations we want to do,” says Fellows.
Many groups are focusing on an alternative strategy that uses electricity, rather than pressure, to do the work of separation. In this technique, an electric current is used to pull dissolved salt ions across specialized ion-exchange membranes, which permit the movement of ions in one direction only. As the ions pass through these membranes, the brine they started out in becomes more dilute, or fresher. Researchers expect the technique to be useful for pre-diluting extremely salty brines so that conventional reverse osmosis can then be used to squeeze out more fresh water.
In one variant of these electricity-based techniques, Lin’s team tried to let the concentrations of salt ions that have crossed the ion-exchange membranes build until they form solid crystals3. This attempt to crystallize salts without evaporating the water worked well for certain salts — such as sodium sulfate, which is commonly found in power-plant waste water, Lin says — but not for the most abundant salt in waste brines, sodium chloride. Sodium and chloride ions hold on to water molecules so tightly that they also drag the water across the membrane, Lin says.
To avoid both evaporation and the use of membranes, Yip’s team members at Columbia are instead looking to chemical solvents4 (see video). One promising candidate is an off-the-shelf solvent called diisopropylamine5. The solvent floats on top of a salty brine and — at low temperatures — selectively sucks water molecules into itself, leaving most of the salt ions behind. At higher temperatures, diisopropylamine switches to repelling water and spontaneously expels the water that it has absorbed, so the water can be recovered and the solvent reused.
Yip says his team has used this method to recover fresh water from brine samples that are up to ten times as salty as seawater — an impossible task for standard reverse osmosis. The fresh water portion might not be potable until further steps are taken to remove contaminant solvent and salt, the researchers say. But the technique could aid industries that are seeking to recycle water from their waste brines. The researchers are currently participating in a prize challenge organized by the US Department of Energy to build a small pilot that would use solar heat for the water-expelling step.
Ren and his colleagues have taken an entirely different approach6, inspired by trees. Trees can draw water up several metres against gravity, emitting clean water vapour from their leaves while trapping dissolved compounds in their tissues. His team's approach mimics trees by using long strings of fibres with one end soaking up salt water. As the brine travels upwards, the salts are separated leveraging the common principle of chromatography — different compounds move at different speeds through a medium.
Ren’s main target, lithium chloride, is extremely soluble and small, so its ions move quickly up the string, ahead of larger sodium ions. Ren has successfully used this method to recover lithium from natural brine samples from Chile, using less energy and space than conventional evaporation. The team is designing an enclosed module incorporating stacks of these strings. The researchers aim to extract lithium from waste brines produced from oil and gas operations, while recovering the evaporated water.
Yet more inspiration could be found in nature: highly-selective channel proteins embedded in cell membranes. One type of ion channel allows just one sodium ion to pass through for every thousand potassium ions, says Elimelech. His team is currently working on membranes that mimic these channel proteins, although for now they are in the earliest stages of development.
Price barrier
Whether any of these ideas will take hold depends on economics. If the SWCC mined all the available sodium chloride from Saudi Arabia's seawater desalination brines, Fellows notes, it would be enough to supply one-third of the world’s market. Meanwhile, waste brines left over from brackish-water desalination could offer the plentiful mineral gypsum, but it’s unlikely that unconventional brine mining could compete economically with conventional quarrying from rock.
New markets, such as the advent of salt-fuelled technologies including zinc–bromine batteries, could create fresh demand for certain salts, says Fellows. Regulations could also play a part, either by making it more expensive to dispose of waste brines or by incentivizing the use of brine-sourced salts in various applications, for instance brine-sourced gypsum in road salts.
One thing is clear: freshwater needs are increasing. Addressing the current limits of desalination with new technologies is important, researchers say. But it isn’t an alternative to the still-essential step of conserving fresh water. It will always take energy, time or land space to separate salt from water, so there will always be a price to pay for desalination. “There’s no magic there,” says Elimelech.
doi: https://doi.org/10.1038/d41586-024-02073-6
This story originally appeared on: Nature - Author:XiaoZhi Lim